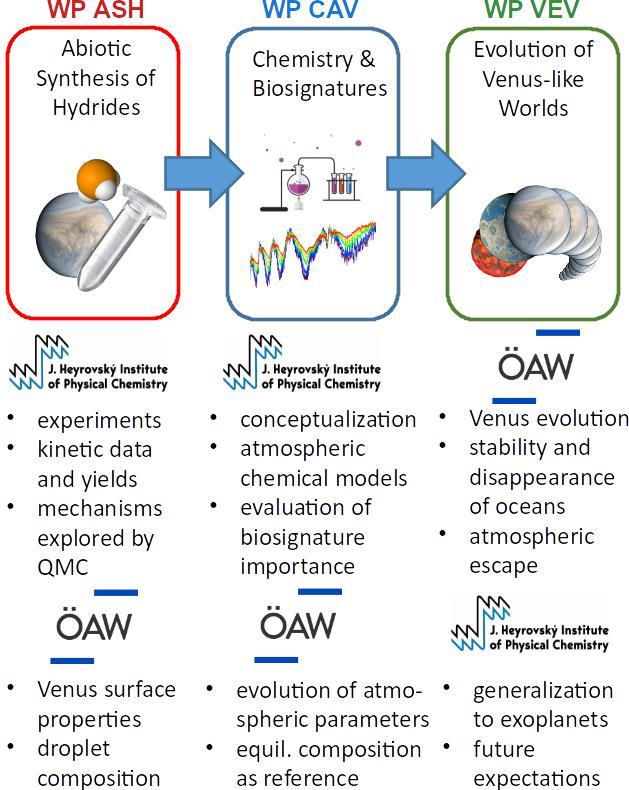
WP ASH: Abiotic Synthesis
Understand the abiotic production of redox disequilibrium pairs
The core of this WPl is a series of novel experiments that will likely convert a redox-neutral phosphorus-containing species into phosphine and some oxidizing species. The unknown is whether this abiotic source of redox disequilibrium is efficient enough to result in detectable abundances of products, and if so, under what conditions.
In general, UV reduction of an oxide in the presence of a source of hydrogen leads to the creation of its counterpart hydride, which is reduced, and another species, typically a mineral, that is oxidized, a redox disequilibrium pair (RDP). We have successfully applied this principle to produce CH4 and perchlorates at Mars, and we intend to test this principle for a Venus-analogue environment to see if our experiments produce the RDPs observed in the Venusian clouds. Showing this in the lab would demonstrate the first example of an abiotic source of redox disequilibrium in an atmosphere requiring a reassessment of how biosignatures, even pairs of biosignatures, should be interpreted.
The efficiency of heterogenous photochemical synthesis of other hydrides (PH3, NH3, and H2S) like the origin of CH4 over acidic surfaces must be explored, and we have to answer the fundamental question: Is this likely or not? Particularly for PH3, we already predicted the feasibility of this process through quantum chemical calculations. We will perform similar calculations to explain the observed chemistry in the Venusian atmosphere and to inform the experimental design. The question above is one of the central topics of this project and the answer can be both positive, partly positive, or fully negative.
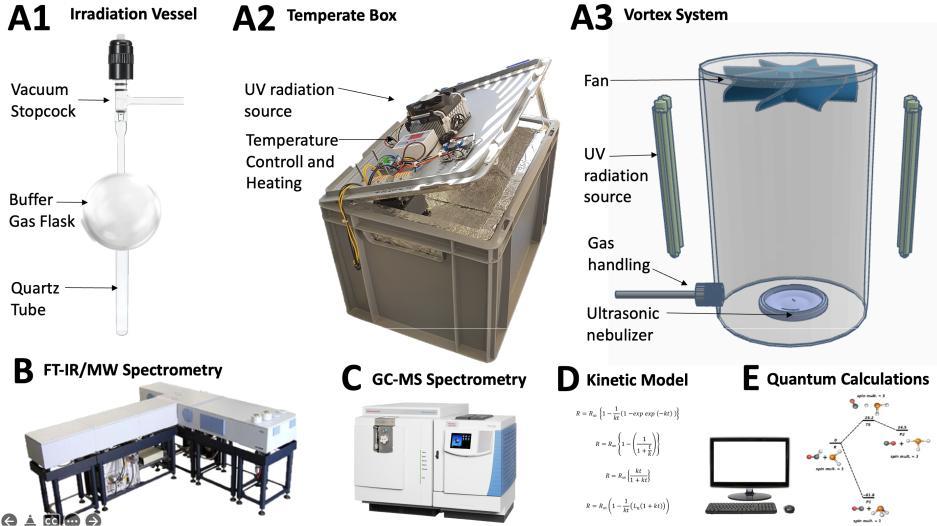
WP CAV: Chemical Modeling of the Venusian Atmosphere
Understand the chemistry of the Venusian atmosphere and clouds
We will convert the experimental yields of PH3, NH3, H2S, CH4, and O2 as provided by WP ASH along with the UV intensity of the laboratory light source to UV-dependent effective chemical rates. Based on these rates we are developing a chemical model for the Venusian atmosphere that also accounts for the distributions and properties of the cloud particles as these are essential for the chemistry of the Venusian atmosphere. This full atmospheric model will be built on the atmospheric model of Rimmer et al. (2021), and should be able to predict the concentration of atmospheric species, specifically of the observed and investigated RDPs. Eventually, these concentrations can be compared to previous observations and predictions.
Parallel to these studies, we will expand the thermo-chemical and phase equilibrium code GGchem to include aqueous chemistry and predict the solution of molecular anions and cations in liquid water. This will allow us to investigate the chemistry in the cloud droplets and at the interface between an ocean or a rocky surface and the planetary atmosphere. Using such an updated version of GGchem, we can scrutinize how far redox pairs of certain molecules – those that are often discussed as biosignatures – can already exist in chemical equilibrium under certain circumstances. We will also use this updated code to better characterize the pH value and availability of nutrients in the water droplets as a precondition for the existence of life as we don’t know it, given the conditions on the ground and in the atmosphere.
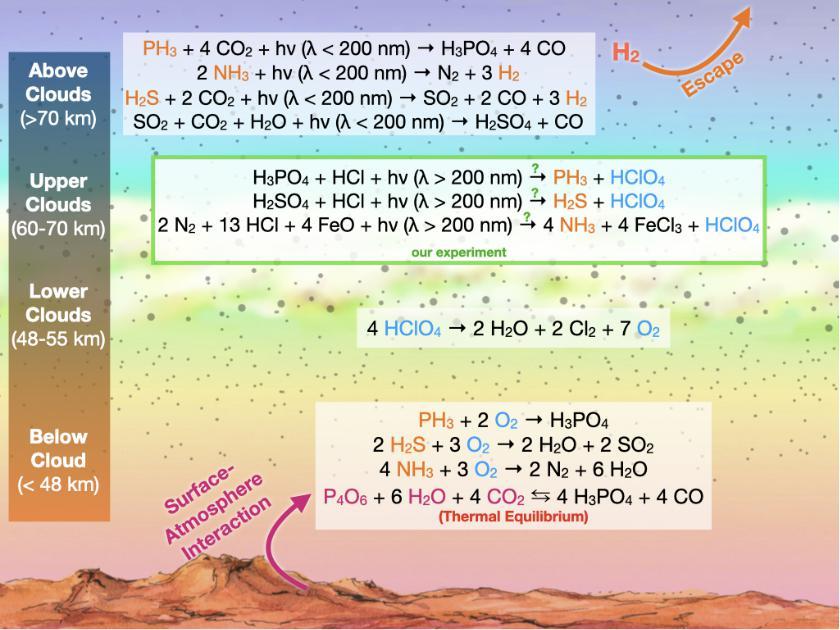
WP VEV: Water Escape from Venus’ Past and Present
Understand the evolution of the Venusian Water Inventory
Venus took an evolutionary pathway that resulted in a very different outcome than the Earth’s. Understanding its evolution is hence key to understanding the emergence and evolution of so-called Earth-like Habitats within and beyond the Solar System. For Venus, the fundamental question to be resolved is whether a habitable environment existed in its past – or even at present. Two different evolutionary scenarios have been proposed in the past for Venus:
- Venus became hot and lost its water early on. It was hence never habitable for life as we know it.
- Venus cooled early on, attained an ocean, and remained temperate and habitable until a resurfacing event made Venus largely uninhabitable, potentially as recently as 700 million years ago.
In these scenarios, the ancient, hypothetically wet and habitable Venus at some point, faced a runaway greenhouse effect that finally transformed the planet into an extremely hot and dry body as the solar luminosity increased. While the water vapor should have mostly escaped to space, a temperate cloud layer containing droplets of H2O with slowly rising sulphuric acid concentration could have potentially formed and survived until the present day. Such a layer could provide habitable conditions for microbial life that migrated from the evaporating water reservoirs into the clouds when the planet evolved from a habitable into the harsh environment we observe today. Even this scenario poses seemingly insurmountable challenges to life as we know it, given that the water activity in the clouds of Venus is two orders of magnitude below the limit for known extremophiles.
To solve this riddle, we will investigate the following issues that are directly linked to the question before:
- Was Venus originally endowed with as much water as Earth, and if so, where did the H2O go?
- What triggered the catastrophic runaway greenhouse effect, how did the composition of the surface and the atmosphere change, and what was the impact on Venus’ surface morphology?
- How did the conditions within the Venusian cloud layer change over time, particularly given the H2O-SO2 fraction?
The results obtained within this project will shed new light on the fundamental question: Was the Earth’s inner neighbor once suitable for the appearance of life, and if so, when and how did its conditions become unfavorable?
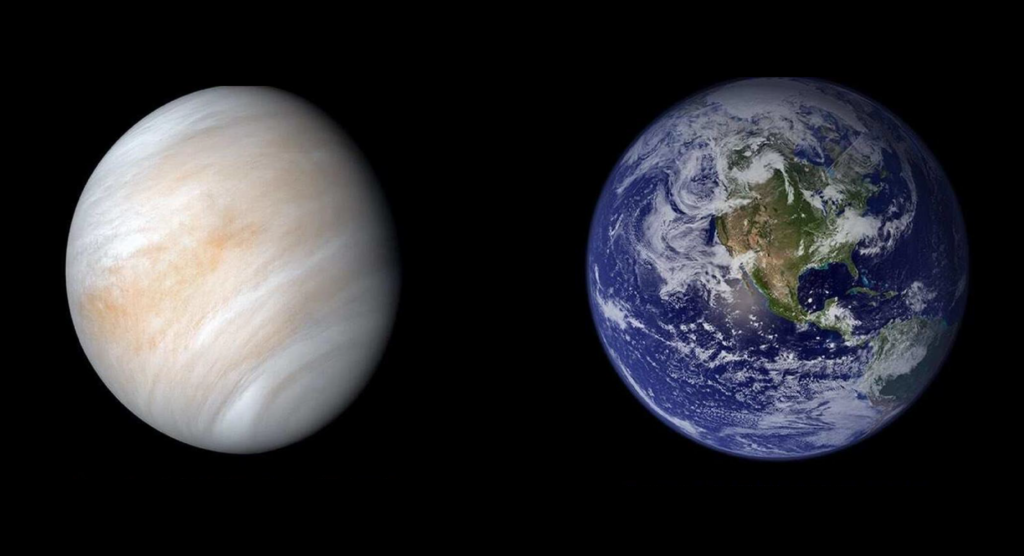